With ten years of experience in additive manufacturing applied to complex products, particularly heat exchangers, SOGECLAIR Aerospace established a consortium to attack this project. SOGECLAIR is a high-tech engineering company in the aeronautical field. They lead the consortium, composed of AddUp, an additive manufacturer, TEMISTh, and the Von Karman Institute for Fluid Dynamics (VKI).
February 2017: among the many topics of the European Cleansky2 Call for Projects, one of them is attracting the attention of many entities, companies, and laboratories. Proposed by Liebherr Aerospace, it concerns the evaluation of improvements in new-generation heat exchangers using additive manufacturing.
With ten years of experience in additive manufacturing applied to complex products, particularly heat exchangers, SOGECLAIR Aerospace established a consortium to attack this project. SOGECLAIR is a high-tech engineering company in the aeronautical field. They lead the consortium, composed of AddUp, TEMISTh, and the Von Karman Institute for Fluid Dynamics (VKI). AddUp is a French industrial company specializing in metal additive manufacturing, TEMISTh is a French developer and supplier of customized thermal solutions, and VKI is a Belgian fluid mechanics laboratory.
SOGECLAIR’s consortium is working on project NATHENA, an acronym for New Additive manufacTuring Heat ExchaNger for Aeronautic. The project will last for four years with a total budget of €1.5M and will be 100% funded by the European Commission. Start date: March 2018.
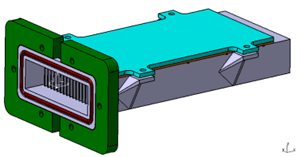
CAD of a single flow channel and interfaces to the test bench
The objective is to develop two innovative heat exchangers for the aeronautical industry. The first one is a “pre-cooler,” allowing the pre-cooling of hot air taken directly from the turbo-engines of an airliner. It will be designed in Inconel 718 because it is subjected to very high temperatures. The second is a “cooler”, located further downstream in the air conditioning chain of the aircraft, allowing the air to be cooled again for later use. It will be designed in Aluminium AlSi7Mg, which performs well at the temperature range in the downstream location. The project aims to design heat exchangers as efficient as those made by conventional methods but with reduced mass and volume.
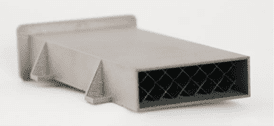
The first step of the NATHENA project is to establish the state of the art of heat exchangers from the point of view of design, numerical simulation, optimization, bench testing, and the associated manufacturing techniques. This work allows us to build a solid database, a structuring element to guide and refine the architectural choices and the geometrical parameters of the future intensification structures developed and characterized during the project. These structures allow for an increase in thermal exchanges by extending the exchange surface.
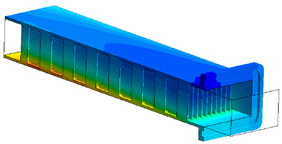
The early technical studies led to the collaborative creation of the first CAD (Computer Aided Design) team of new intensification structures. To select the most promising geometries, the aim is to estimate their performance, factoring in manufacturing, mechanical, fluidic, and thermal perspectives. These are then integrated into the project’s standardized test channels, printed in Inconel and Aluminium on an AddUp’s machine, the FormUp® 350.
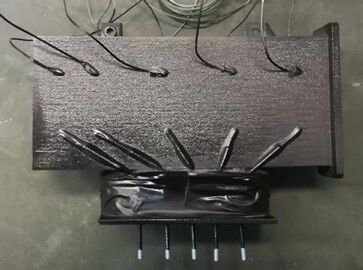
Instrumented single flow channel
Less than ten channels are printed in each material, one channel per intensification structure. These channels are then thermally tested on a test bench, and the experimental results are compared to CFD numerical simulations (Computational Fluid Dynamics). The principle: air at room temperature is introduced at the entrance of the channels while the heat exchangers are heated by a flat electrical resistor attached to one of their walls. Multiple sensors then measure the gas’s pressure, temperature, and velocity at different positions in the channels. These measurements are then used to confirm the validity of the numerical simulation models and to compare the performance of the different structures.
Through simulation and testing, the consortium gains a better understanding of the flows and heat transfers in different structures produced by additive manufacturing. The manufacturability of such geometries with many thin walls is evaluated as well. These first very encouraging results allow us to outline the most efficient heat exchanger architecture, offering the best compromise between manufacturability, mechanical strength, thermal performance, and fluid performance. All these results continue to build on the already established database.
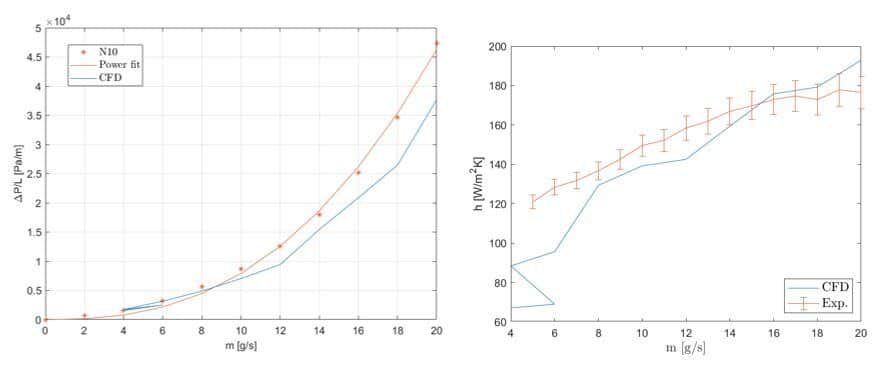
This first study on the representative channels thus launches a campaign of similar tests involving two hot and cold fluids. The goal is to characterize the performance of the chosen geometry in a miniature heat exchanger in which the hot source is no longer an electrical resistor but a hot air flow. The channels here will be in a crossed configuration.
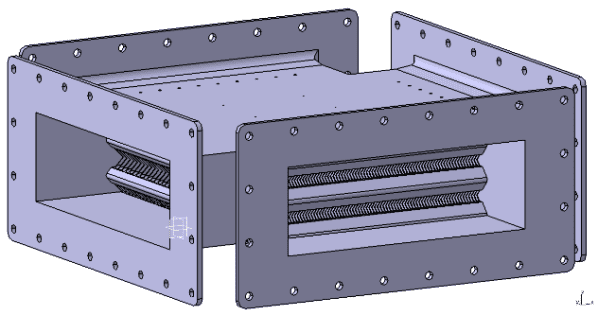
Three two-fluid channels are printed: two in Inconel and one in Aluminium, for which the parametric intensification structures are calculated and adapted according to the airflow characteristics. The manufacturing of a very large number of thin-walled fins (several thousand), with the associated printing quality, dewaxing, and finishing requirements, is a real challenge. The channels are then characterized on a test bench, allowing once again to correlate experimental tests and numerical simulations.
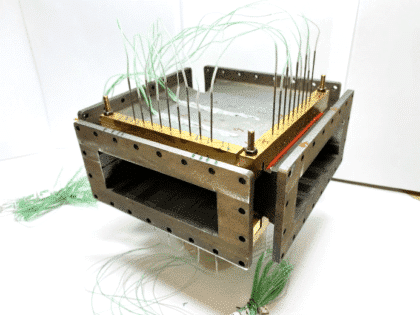
Instrumental dual flow channel
Thanks to a homogenization method, these thermo-fluidic characterizations have enabled the creation of metamaterials (“Equivalent Porous Media” or EPM with equivalent volume properties) which simplify the numerical simulations, lighten the models, and reduce the calculation time. Correlations between numerical simulations and tensile tests of specimens, also produced in Inconel 718 and Aluminium AlSi7Mg, allow us to refine these mechanical metamaterials. Indeed, a heat exchanger is a system with numerous small and complex geometries in a large volume. Simulating them numerically can be very computationally expensive if such techniques are not used.
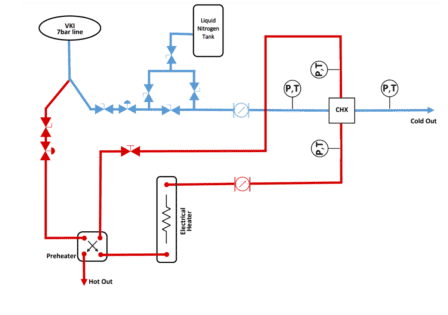
The next step is to integrate the most efficient intensification structure in two larger prototypes of heat exchangers (one in AlSi7Mg and one in Inconel 718). As in the previous step, the objectives are to improve performance and better correlate the results between numerical simulations and experiments on the test bench. The aim is to generate as much data as possible and to increase the knowledge of additive manufacturing applied to complex thermal equipment.
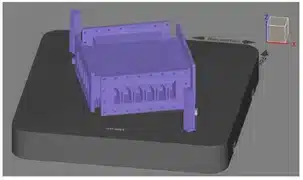
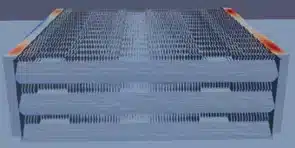
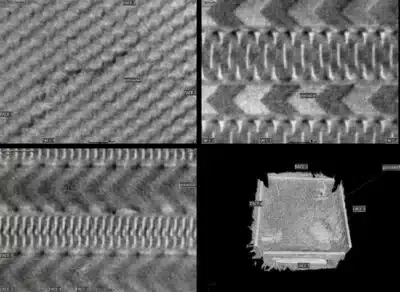
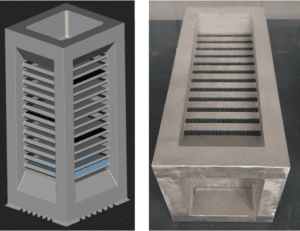
All these simulations and experiments have enabled us to precisely determine the performance of the selected intensification structure and the internal architecture. The two final heat exchangers were designed to meet Liebherr Aerospace’s specifications accordingly. New tools and innovative methods had to be used to realize their CAD, considering the size of the designs and the very high number of integrated intensification structures (more than a million). The data generated by the test of the Aluminium prototype was also used to simulate the theoretical performance of the final exchanger and to generate a first CAD of the full part, with a volume corresponding to 12 printed prototype cells (see below).
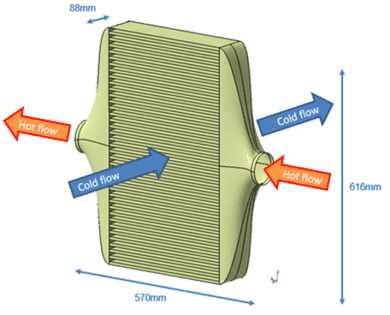
In order to meet the performance requirements of the final part, a focused study was carried out based on the initial data from AddUp and adapted to the specific needs of this project. The specifications expressed by the consortium contain 3 major points:
- Thin waterproof walls in IN718 (between 100 and 300µm)
- Productivity increase
- Surface finish on fins and channels <6µm
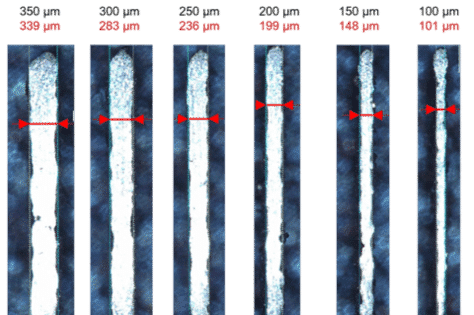
To master the constraints of this development, AddUp uses the latest generation machine (FormUp 350 – New Generation) allowing the use of 4 lasers as well as enhanced monitoring and tracking systems (sensor monitoring, recoating control, …). This data, coupled with the results of experimental measurements, have made it possible to define an operating range and a set of stable manufacturing parameters.
The complete heat exchanger was produced with a manufacturing strategy that allowed the simultaneous use of 4 lasers to increase the productivity of the laser powder bed fusion technology. This performance was made possible by the prior validation of the various key characteristics of the part (mechanical, thermal, dimensional).
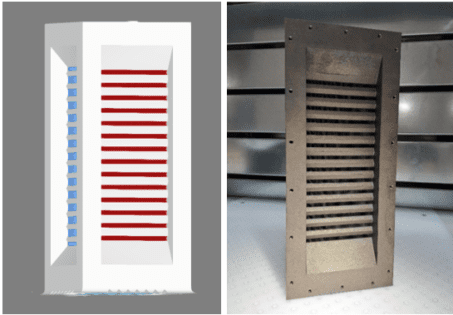
Like the Aluminium exchanger, the Inconel exchanger was tested on a test bench to evaluate and validate the first models established for Aluminium. These experiments allowed us to study and highlight the impact of the roughness, but also to validate the first behavior models used during the simulations.
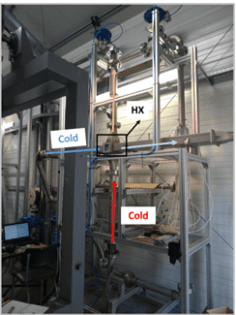
The roughness-related deviation is considered in the heat flow simulations performed by Temisth. The calculations show a temperature distribution matching the data from the real measurements, validating the first models used.
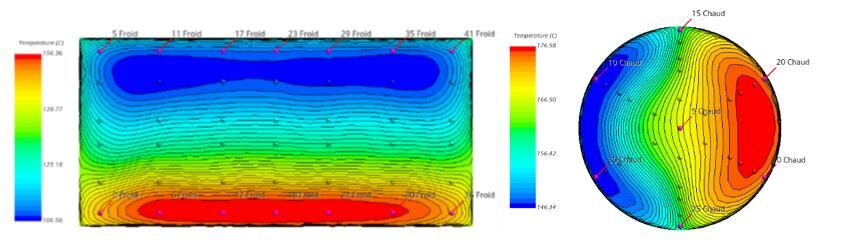
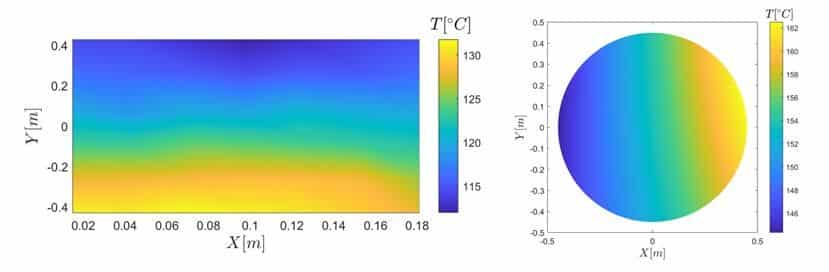
The size and details of the final complete exchanger (670x450x320mm) in Inconel 718 from the conclusions of the study show the possibility of integrating additive manufacturing for the realization of heat exchangers with performances at least matching that of current heat exchangers.
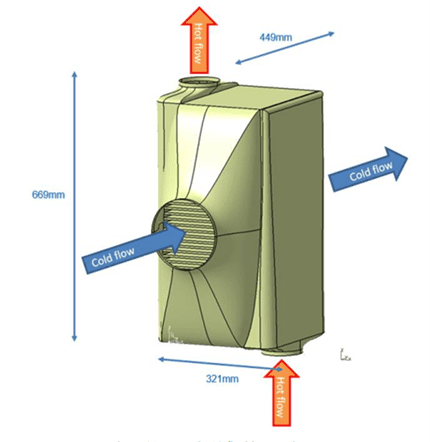
Technological hurdles overcome within the project :
- Depowdering
- Manufacturing strategy for thin walls
- Manufacturing strategy to reduce surface roughness
- Generation of a high air flow at -15°C
- Temperature measurement mapping
- Correlation between simulations and experimental measurements
- Calculations based on experimental measurements to predict the aerothermal performance of fabricated exchangers
- Management of large files
- CAD methodology adapted to complex structures
- Calculation methodology adapted to complex structures
- The manufacturing strategy allows the use of 4 lasers on the same part
NATHENA: the consortium
SOGECLAIR aerospace
With its roots in aeronautics, the SOGECLAIR group designs, manufacture, and supports innovative solutions and products for transport in the civil and military fields.
Its R&D policy supports its participation in major future programs such as the development of the aircraft of the future and autonomous vehicles.
Its subsidiary, SOGECLAIR aerospace, is an international leader in the design and integration of high-value-added solutions for the aerospace industry. It designs, manufactures, and maintains the main components of aerostructures and aircraft interiors.
SOGECLAIR aerospace develops and deploys advanced materials and technologies such as thermoplastics and additive manufacturing.
With more than 1600 employees worldwide, SOGECLAIR aerospace has recognized know-how in:
- Design and architecture of aerostructures and systems,
- Design and manufacture of aircraft interiors,
- Configuration management at the program, engineering, and industrial level,
- Design and manufacture of simulated and embedded equipment.
AddUp
AddUp, created in 2016, is a joint venture between Fives and Michelin. It is a provider of complete industrial metal 3D printing solutions.
AddUp is involved in:
- Design and manufacture of machines integrated into a complete production line, from powder management to the finished part,
- Customer support for the production of metal 3D printed parts, to support investment projects in additive manufacturing for aerospace or additional production needs,
- Cross-functional service activity, including the redesign of parts and additional services associated with the machine offering, helps companies find the most appropriate technical and financial solutions.
TEMISTh
TEMISTh is a company that specializes in developing and supplying customized thermal solutions. To do so, the company develops numerical simulation and optimization tools for automated heat exchanger design. This allows the company to develop new heat exchanger concepts to be produced by additive manufacturing.
Thanks to its location at the TEAM Henri Fabre Technocentre, TEMISTh offers various advanced manufacturing technologies such as metal and polymer additive manufacturing, foundry, machining, and assembly for function hybridization through brazing or friction welding. TEMISTh’s mastery of all these processes enables it to offer optimized and successful solutions to all its customers. All parts developed and produced can then be tested on TEMISTh’s thermal test benches.
The industrial fields in which the company operates are numerous: aeronautics, space, transport, oil and gas, and electronics.
Von Karman Institute for Fluid Dynamics
The Von Karman Institute for Fluid Dynamics (VKI) was founded in 1956 by Professor Theodore Von Karman as an international center combining education and research for the citizens of NATO countries under his motto “Training in research through research”.
Educational programs provided: Conferences / Courses / Colloquia, Short Courses, Master’s Thesis, Master of Research in Fluid Dynamics, Doctoral Program, and Applied Research Program.
The VKI undertakes and promotes research into experimental, computational, and theoretical aspects of liquid and gas flows in the fields of aeronautics, aerospace, turbomachinery, environment, and industrial and safety processes. Some 50 specialized test facilities are available, some of which are unique or among the largest in the world.
Research is conducted under the direction of faculty and research engineers, mainly sponsored by governmental and international agencies and companies.
Liebherr Aerospace
Liebherr Aerospace designs, develop, and manufactures air systems, flight control systems, and landing gear, as well as gears and gearboxes, and electronics for the aerospace industry. Liebherr Aerospace provides complete OEM customer services through a global network that offers equipment repair and overhaul, technical support and documentation, spare parts supply, and AOG service.
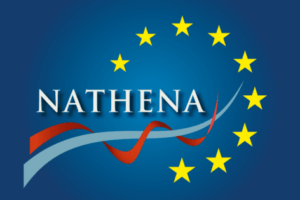
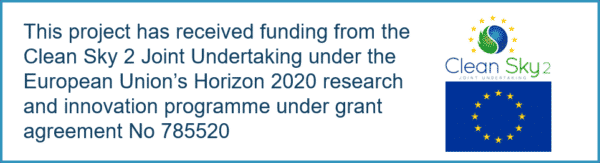